How genetically engineered mice (GEM) were generated prior to CRISPR-Cas9 technology
The use of CRISPR-Cas9 technology offers a convenient and cost-effective method to generate various transgenic mice that have frame-shift mutations, point mutations, gene knock-outs and gene knock-ins for biomedical research. Before CRISPR, transgenic mice were generated by pronuclear microinjection (PNI) of an exogenous DNA cassette (foreign genetic material) into a one-cell stage mouse zygote [1]. This approach resulted in random integration of the transgene into the mouse genome. The efficiency of the method was low and random integration could lead to unpredictable and potentially harmful off-target effects. A more precise method was developed using homologous recombination in embryonic stem cells with a gene targeting vector. In this method, engineered embryonic (ES) stem cells carrying the gene-targeted mutation are first injected into the blastocyte stage embryos which are then transferred to a host mother [2]. The F0 mice born from the embryos are chimeras which contain tissues originating from both the ES cells and the recipient embryos. Chimerism is physically noticeable due to the coat color difference between the strains of ES cells (e.g., brown) and the host blastocyte (e.g., black). The next F1 generation mice were bred to obtain a mouse strain that carries the desired gene mutation in the germline (solid brown coat color).
Advantages of using CRISPR tools to generate mouse models
These traditional ways of creating transgenic, and knock-out or knock-in mouse models were time-consuming, needed sophisticated equipment, and highly skilled technicians. Advancement in gene editing with CRISPR-Cas9 technology offers the ability to create mouse models in an efficient and prompt manner. This technology includes the delivery of a Cas9 nuclease which is the enzyme that creates the double strand break (DSB) in DNA and a guide RNA which directs the Cas9 nuclease to the target region within the genome into one cell stage mouse embryo using PNI (Figure 1). To generate knock-out mice, the Non-Homologous End Joining (NHEJ) mechanism repairs the DSB by introducing an insertion or deletion (indel) mutation [3]. For generating knock-in mice, the homology direct repair (HDR) mechanism repairs the DSB using a donor repair DNA template [3]. Generating double mutant mice is also possible by designing two guide RNAs to target two target genes.
Besides the PNI approach, CRISPR components can be delivered using viral vectors, such as adeno-associated virus (AAV) or via electroporation directly to many zygotes at one time [4]. In fact, researchers can deliver CRISPR reagents into zygotes via electroporation within the mother through a method called Genome Editing via Oviductal Nucleic Acid Delivery (GONAD) [5]. A more efficient approach is electroporation of CRISPR/Cas9 delivered as a ribonucleotide protein (RNP) to zygotes followed by the injection into the host mother [6].
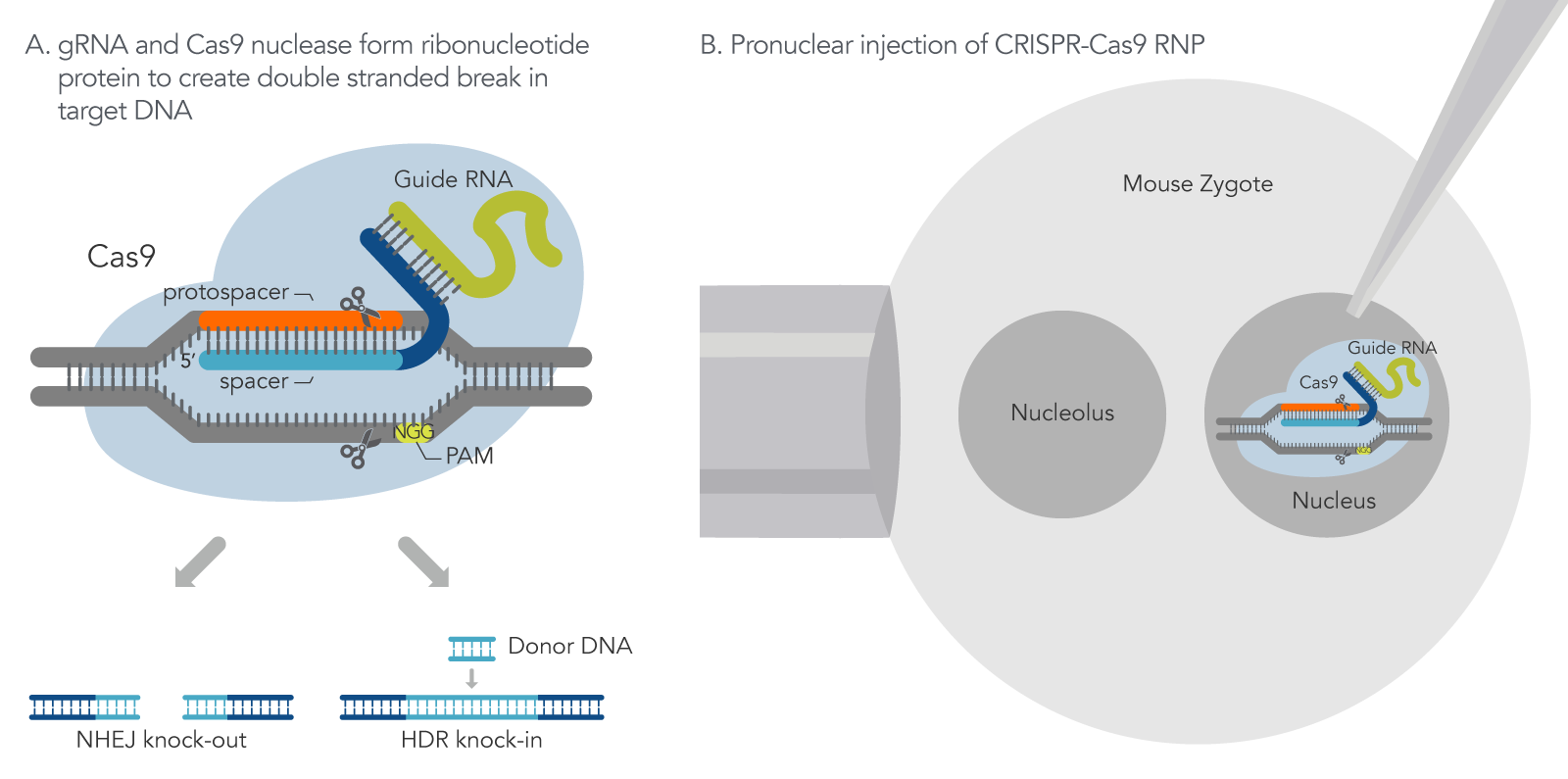
Figure 1. How CRISPR-Cas9 technology is used to generate mouse models. (A) With CRISPR-Cas9 gene editing, the guide RNA directs the Cas9 nuclease to make a double strand break (DSB) at a target region within the genome. Depending on the guide RNA design, Cas9 makes a DSB at the target region to either disrupt a gene-coding region (knock-out) via the non-homologous end joining (NHEJ) repair mechanism or remove a gene-coding region with a replacement of a repair DNA template via Homology Direct Repair (HDR) mechanism (knock-in). (B) CRISPR-Cas9 single guide RNA (sgRNA) and Cas9 nuclease are injected into a mouse zygote.
Alternative methods using CRISPR tools to generate mouse models
Creating conditional mouse lines such as CRE/LoxP mice are useful for studying gene activation or repression. With CRISPR technology, LoxP sites can be inserted into the mouse zygotes, and the F0 mice are mated to establish the line. For tissue or cell specific genome editing, conditional mouse models can be generated to study gene activation and repression within specific tissues such as the brain and liver [7].
Besides generating mouse model lines starting with embryos and transferring the engineered embryos to foster mothers, and breeding, CRISPR technology is applicable for performing direct genome editing in vivo in mature mice. This is done by identifying gene mutations underlying a disease then designing and injecting an adeno-viral vector (i.e., associated virus (AAV9) vector) containing CRISPR components. Multiple studies have been reported to demonstrate these alternative approaches by using direct CRISPR-mediated gene editing to study various human diseases including cancer, diabetes, muscular dystrophy, acute kidney disease, and herpes simplex virus (HSV) [8-11].
Get started with CRISPR
The advancement of genome editing using CRISPR-Cas9 technology allows for scientists to generate transgenic animal models more efficiently and quickly. With CRISPR-Cas9 tools, researchers can produce useful and reliable knock-out, knock-in, and double mutant models with protein tags, fluorescent reporters, and loxP sites within targeted genes to study various human diseases in the laboratory.
Excited about taking the next step in applying CRISPR in your laboratory? Learn more about CRISPR-Cas9 technology and the essential tools you need to succeed in your CRISPR experiments, read more about gene editing in our CRISPR handbook.